Revealing the Thermal Noise Caused by Qubit Control Lines
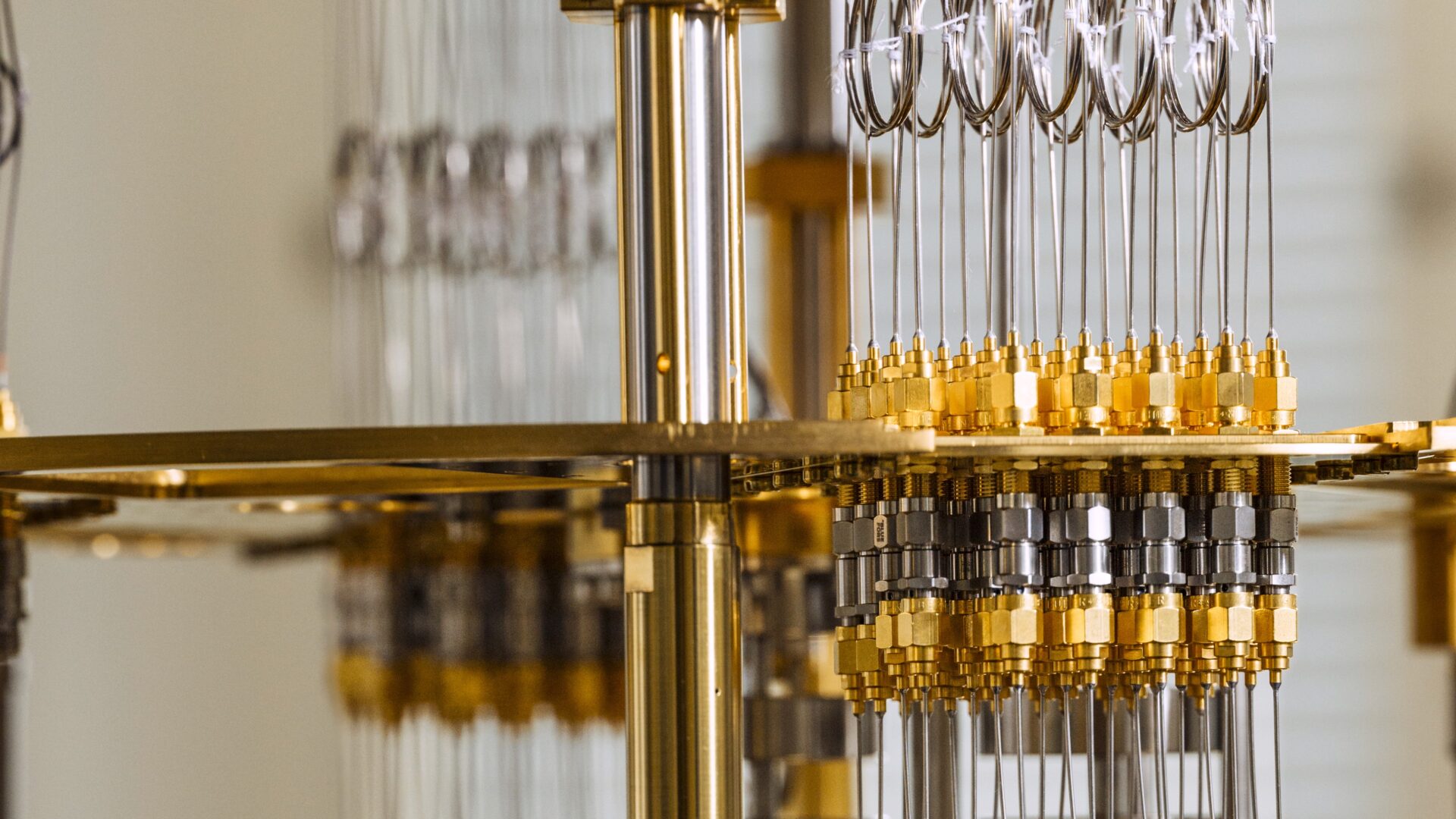
PRX Quantum published a Bluefors research paper, “Inherent Thermal-Noise Problem in Addressing Qubits”, reporting quantitative and device-independent measurements of the power radiated to a quantum processor from its control lines and theoretical predictions on the impact of the measured power on real qubit operations. This research, carried out by Bluefors’ Quantum Applications team, explains the qubit dissipation and decoherence rates observed in previous studies, but also sets the stage for more accurate noise modeling in novel quantum computer interfacing methods thanks to its device-agnostic approach. The paper concludes that thermal noise introduced in the control wiring will not limit quantum computer performance in the near future.
Noise and Qubit Control
Qubits — the building blocks of quantum computers — are famously sensitive to noise. Thermal photons that make their way to quantum chips appear as noise, causing the quantum states of these qubits to decay. For this reason, many of the leading candidates for quantum computer architecture are protected by shielded environments cooled to ultra-low temperatures near absolute zero temperature to limit the effects of thermal noise on qubits. Suitable conditions can be achieved inside Bluefors dilution refrigerators, such as the XLDsl system used in the new study.
This scenario poses the following fundamental question: if the computer is perfectly isolated, how can we send and receive information to enable addressability and qubit-specific measurement?
For qubit control and readout, researchers must “wire up” the qubits, and these wires will transmit signals across more than four orders of magnitude in the temperature gradient to connect directly to the sensitive quantum hardware. The wires must pass through the shielding and plug into the quantum device, introducing extra heat and noise to the system. Even a few excess photons of noise are enough to significantly affect qubit dynamics.
Today, the amount for qubit interconnects in systems is increasing, and the community is racing to establish hardware specifications for fault-tolerant quantum computers. Because of this, characterizing the noise caused by the wiring is crucial. But while the specific problem has been known in the field for a long time, accurate measurements of the radiative heating from cryogenic wiring have been difficult to acquire with conventional power sensing methods.
Revealing the Noise
This new article reports quantitative results on the magnitude of radiative heating introduced by quantum computing control wires. The results were achieved by installing a sensitive thermal detector at the coldest stage of the cryogenic system and calibrating its response with a cryogenic blackbody source. The detector used was a coaxially coupled, ultrasensitive nanobolometer, which was calibrated using a Cryogenic Variable-Temperature Noise Source.
Scientists from Bluefors’ Quantum Applications team performed a systematic study to investigate five varieties of typical qubit control lines that spanned the whole temperature range from room temperature to the coldest part of the cryogenic environment at the millikelvin stage. During measurements, the lines were then connected to the detector one by one. In this way, they accurately recorded both the steady-state power and dynamic properties of the noise that qubits experience under standard operating conditions. The steady-state case corresponds to a practical scenario of thermal noise generated by heavy use of a quantum processor with on average constant microwave power applied to its drive lines, whereas dynamics may come into play when utilization is infrequent.
The experimental results represent a new addition to the literature in the form of a large dataset of noise temperature data for typical drive lines exhibited in unprecedented clarity and accessing the thermodynamical properties of the lines, including their thermal time constants and heat capacities. Previous studies measured noise by observing the degradation of the performance of specific qubit devices. In contrast, the new results in the paper were obtained using a cryogenic power sensor with a high dynamic range that is agnostic to any specific qubit implementation. This means that the data is widely applicable and can be used to interpret many different experiments around the world.
Impact on Qubits & Outlook
The measured thermal latencies of the lines were significantly longer than typical pulse durations in qubit control, and therefore, at present, do not pose an issue to quantum algorithms. The results from the steady-state measurements were fed to a quantum mechanical model that treats the measurement system and quantum device within the holistic framework of open quantum systems. Based on this analysis, the researchers were able to infer the effect of self-heating in the wires during individual control operations on the qubit.
Considering steady-state noise levels, it was found that typical single- and two-qubit control operations are not affected significantly. In other words, such operations can still be performed fast and with high fidelity compared to state-of-the-art, with the caveat that the coupling to the qubit must be selected accordingly.
To summarize, the study shows that commonly used cryogenic wiring components provide a sufficiently low level of thermal noise for near-term quantum computing applications. Furthermore, the results establish an effective method for evaluating emerging technologies to be used inside quantum computers, including flexible wiring, photonic links, or cryo-CMOS electronics.