Utilizing Synthetic Quantum Materials to Study Many-Body Systems
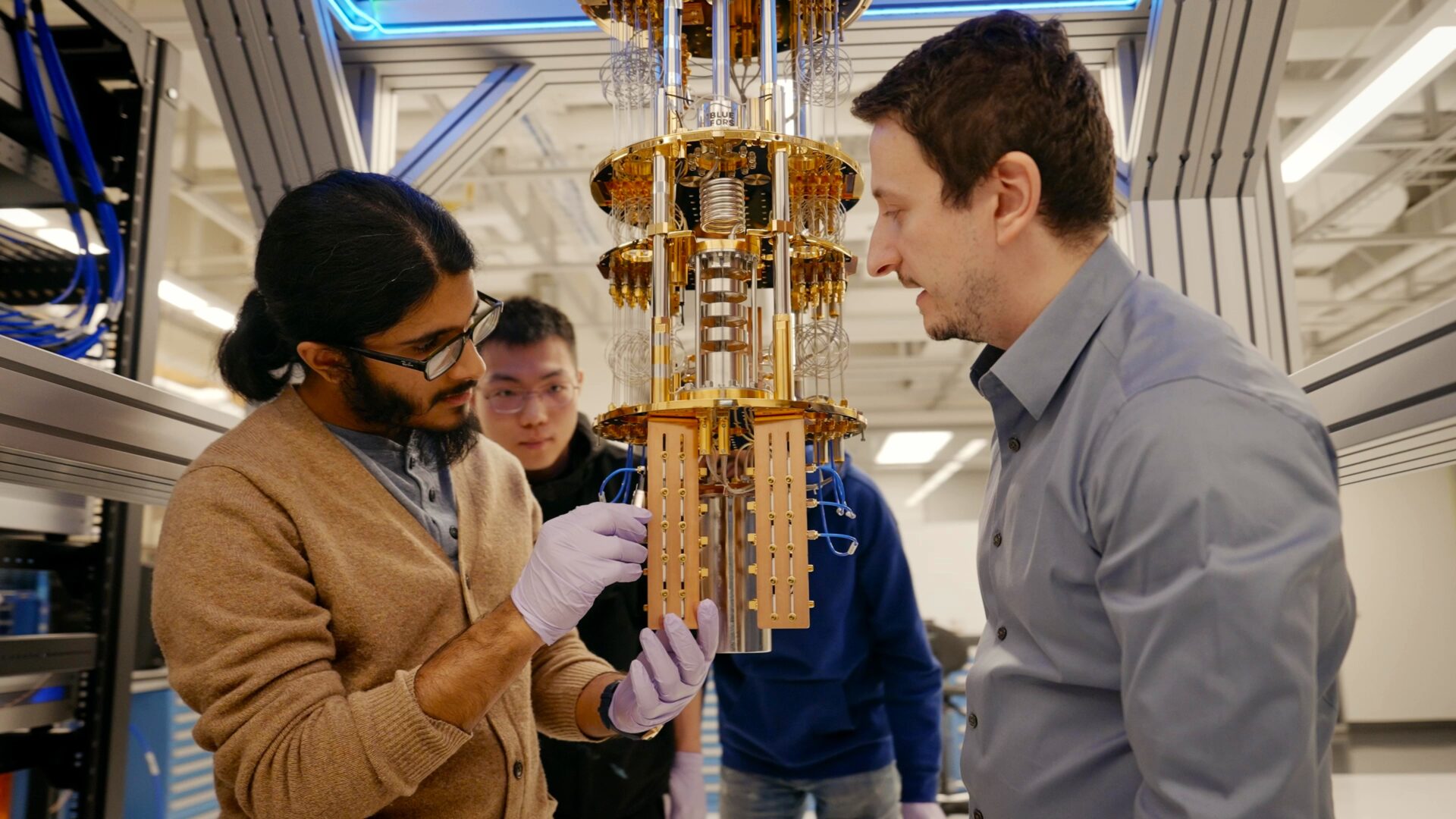
Andrei Vrajitoarea, Assistant Professor at the Physics department in New York University (NYU), heads the Vrajitoarea Lab, which focuses on the development and exploration of synthetic quantum materials.
The team builds synthetic quantum matter from strongly interacting microwave photons using superconducting quantum circuits. These artificial materials, or quantum simulators, provide a unique platform to study many-body physics in a highly controllable way.
In this case story, we explore the knowledge that this type of experimental physics research yields, what recent results have been achieved with this research, and look at the long-term goals of this work. You can also watch an interview with Andrei Vrajitoarea, produced in collaboration with The Quantum Insider.
Exploring Many-Body Phenomena with Interacting Photons
Coming from an electrical engineering background, Andrei became curious about the underlying physics of electronic devices – how they function and enable the processing of information. This sparked an interest in quantum effects that ultimately motivated him to dive deeper into the field of quantum physics.
“What I was particularly fascinated about was that quantum effects started to dominate once we made devices smaller and smaller (to be able to increase the density of computing hardware). This drew me into harnessing these quantum effects and these integrated devices, for applications both in quantum information, as well as in simulating quantum physics,” Andrei says.
Now Andrei heads a research group exploring physics at the interface of condensed matter, quantum information, and quantum optics. The Vrajitoarea Lab investigates many-body physics – the collective behavior of interacting particles – to uncover how microscopic quantum phenomena influence the macroscopic properties of materials. As an example, quantum effects play a key role in materials and phenomena that are vital to modern technologies such as magnetism and superconductors. By advancing our fundamental understanding of these, the research has the potential to drive development in a wide range of technologies.
One of the significant challenges in this field is the computational power needed to simulate many-body problems. The required computational resources increase exponentially with the number of constituent particles, making classical machines insufficient for studying these problems.
“This has motivated my research, as well as the research of many groups around the world, to develop synthetic quantum materials in the lab, where we can explore many-body physics in a highly controllable way using novel control and detection schemes, as well as navigating extreme parameter regimes, beyond what is accessible in conventional solid-state materials,” Andrei explains.
At their lab, the team is building synthetic quantum materials from strongly interacting microwave photons using the experimental toolbox of superconducting quantum circuits. Leveraging the coherent quantum control and flexibility of superconducting circuits provides a versatile platform for assembling photonic materials with complex many-body interactions. By patterning a grid of coupled superconducting qubits, Andrei and the team can engineer an artificial lattice for microwave photons (similar to electrons in a lattice), where the underlying interactions stem from the nonlinearity of the Josephson junction.
“The long-term goal of my research and my group’s efforts is to build synthetic quantum materials from microwave photons exhibiting strong long-range interactions, enabling the photons to self-organize into a highly entangled quantum state,” Andrei explains.
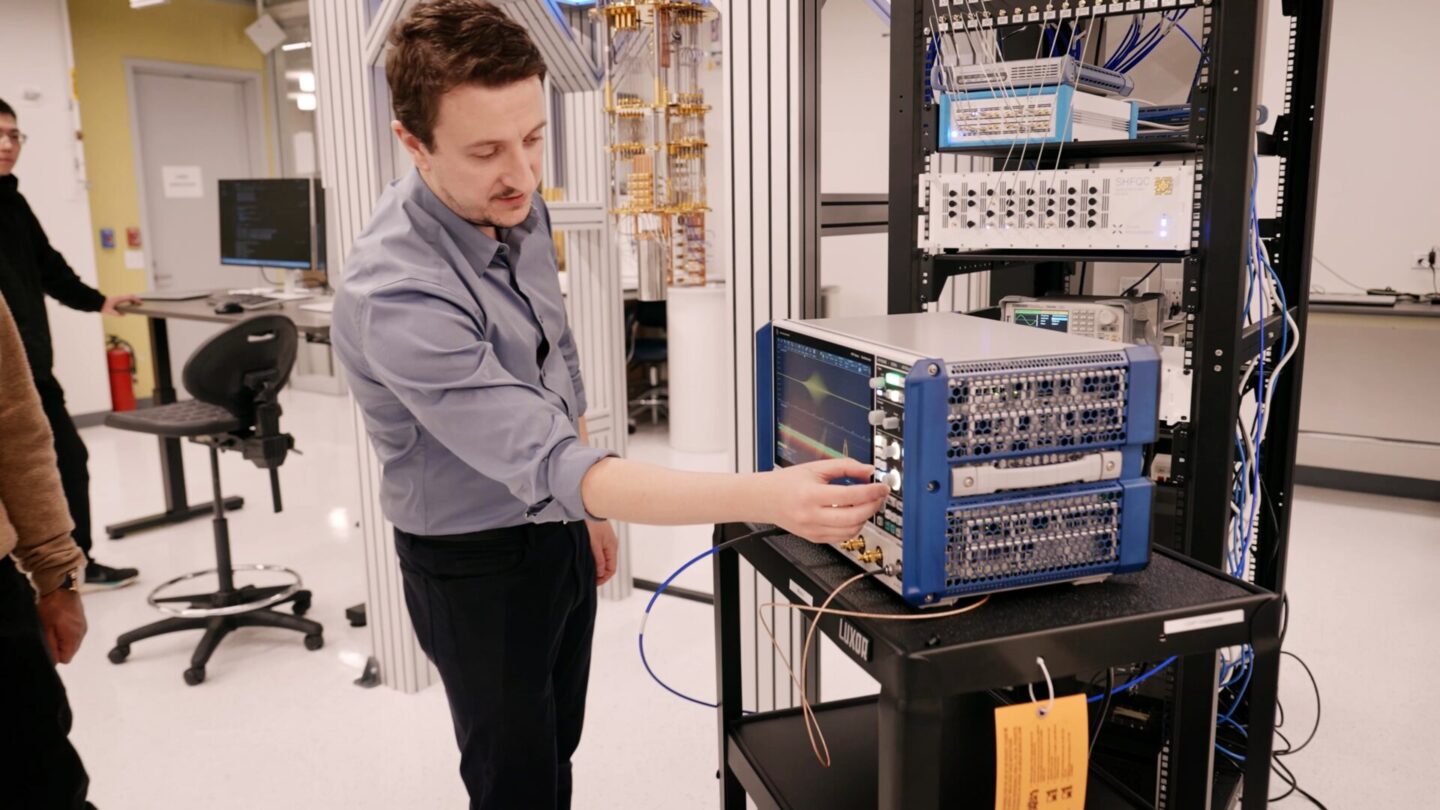
Controlling Quantum Systems
However, building many-body quantum simulators is only one part of the journey – much more focus is needed on controlling them. “When it comes to building these synthetic quantum materials, the main challenge is not necessarily building the platform out of many individual quantum systems and having them interact with each other, but it’s actually guiding this system to a target entangled many-body state.”
When trying to control and probe these systems, researchers are always trying to avoid decoherence. With more efficient control techniques, the researchers can reach their target states faster to avoid these issues.
“The issue with generating these entangled states, and in general evolving our many-body system to get the quantum effects that we want, is that we’re also fighting with decoherence, loss of particles and loss of phase information. This is why it’s important to come up with new techniques for efficiently steering these artificial many-body systems at time scales faster than decoherence,” Andrei explains.
Previously, Andrei and other researchers introduced a new quantum control technique for manipulating synthetic quantum materials. This method allows for the preparation of highly-entangled states by harnessing the intrinsic quantum nature of the platform.
Andrei explains that the standard method for controlling quantum systems involves classical control fields and parameters, and there is much to be gained if instead the control parameters are quantum states. “In this approach, we challenge conventional methods by controlling the dynamics of our many-body quantum system conditioned on the quantum state of our lattice sites (the qubits). Similar to how a transistor uses a classical gate voltage to regulate the flow of electrons across its channel, we envisioned implementing a quantum transistor for photons,” he says.
The researchers were able to achieve quantum control over the transport dynamics in their system. “We found that we can harness the intrinsic quantum nature of our synthetic platform to have genuine quantum control over the dynamics of our many-body system. So if our control qubit was in the zero or one photon state, it will allow our many-body system to evolve in one trajectory or another,” he continues.
Andrei elaborates on the impact of their results: “It allowed us to achieve quantum control over the evolution of our many-body system, allowing us to simultaneously transverse and interfere with multiple trajectories. This interference enabled us to prepare a coherent superposition of different phases of matter. In this case, the synthetic material was prepared in a coherent superposition of photons organized in both a solid and fluid phase. As a direct consequence, we could prepare highly entangled multi-qubit cat states, which would have been difficult to do using conventional classical control of our quantum hardware.”
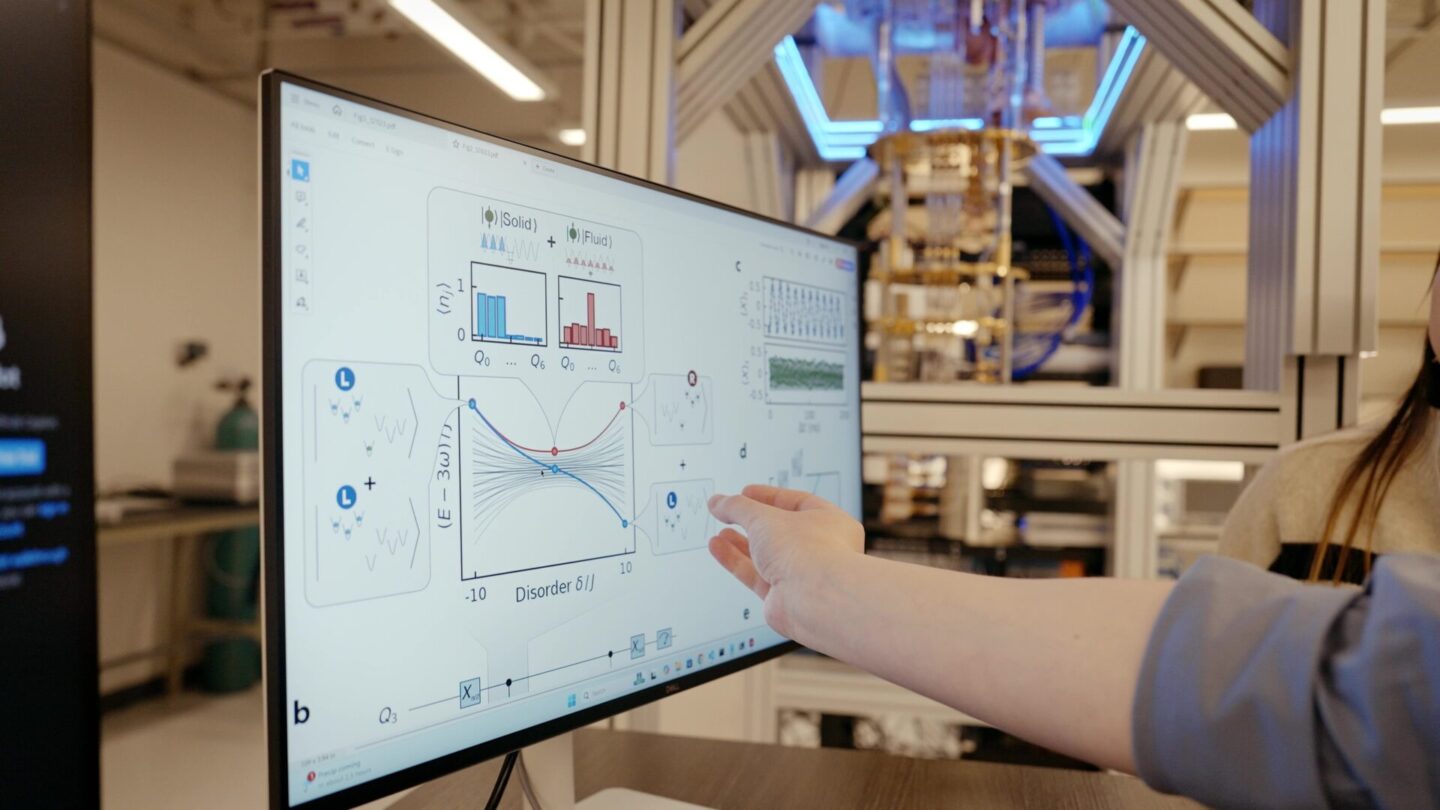
Cooled Down Microwave Circuits
Ultra-low temperatures play a critical role in achieving the required level of control over many-body systems and observing individual quantum states. “Since we’re dealing with microwave circuits that oscillate at around 5 to 10 gigahertz, it becomes important to reach a temperature where the thermal occupation of these quantum circuits is very small. This is why we have to use a dilution refrigerator that can reach temperatures of around 8 to 10 millikelvin,” Andrei says.
To achieve these temperatures, the team utilizes a Bluefors LD400 Dilution Refrigerator in their lab. It is used to cool down microwave circuits and contains additional microwave components for controlling and probing qubits. “In the dilution refrigerator, we have individual microwave lines that are carefully thermalized, attenuated and filtered, delivering signals to control our individual qubits. We have also carefully filtered the microwave components to amplify the very weak signals that are coming out of our devices. Those are used for probing the quantum states of our synthetic platform.”
In their work, the team develops and refines multiple device iterations to create one that matches the required parameters for their research. Once all the parameters are within desired specifications, the team can start exploring science with the multi-qubit devices.
“Once we have a device that we’re ready to do long-term science on, our typical cooldowns can last somewhere around one to two years. So that’s where it becomes also important to be able to have a dilution refrigerator that can reliably maintain that cooling power; that can maintain those temperatures for a long enough time to perform these experiments,” Andrei mentions.
The LD400 system at their lab was installed in 2024, and utilizes the second-generation Gas Handling System. When asked what strikes him the most about the new system, Andrei highlights the new hardware utilized by the system: “For me, the most important change has been changing the pump that’s used for flowing the helium mixture. The new pump is more robust, and it can handle longer usage times without maintenance, which is critical for operating longer time cooldowns.”
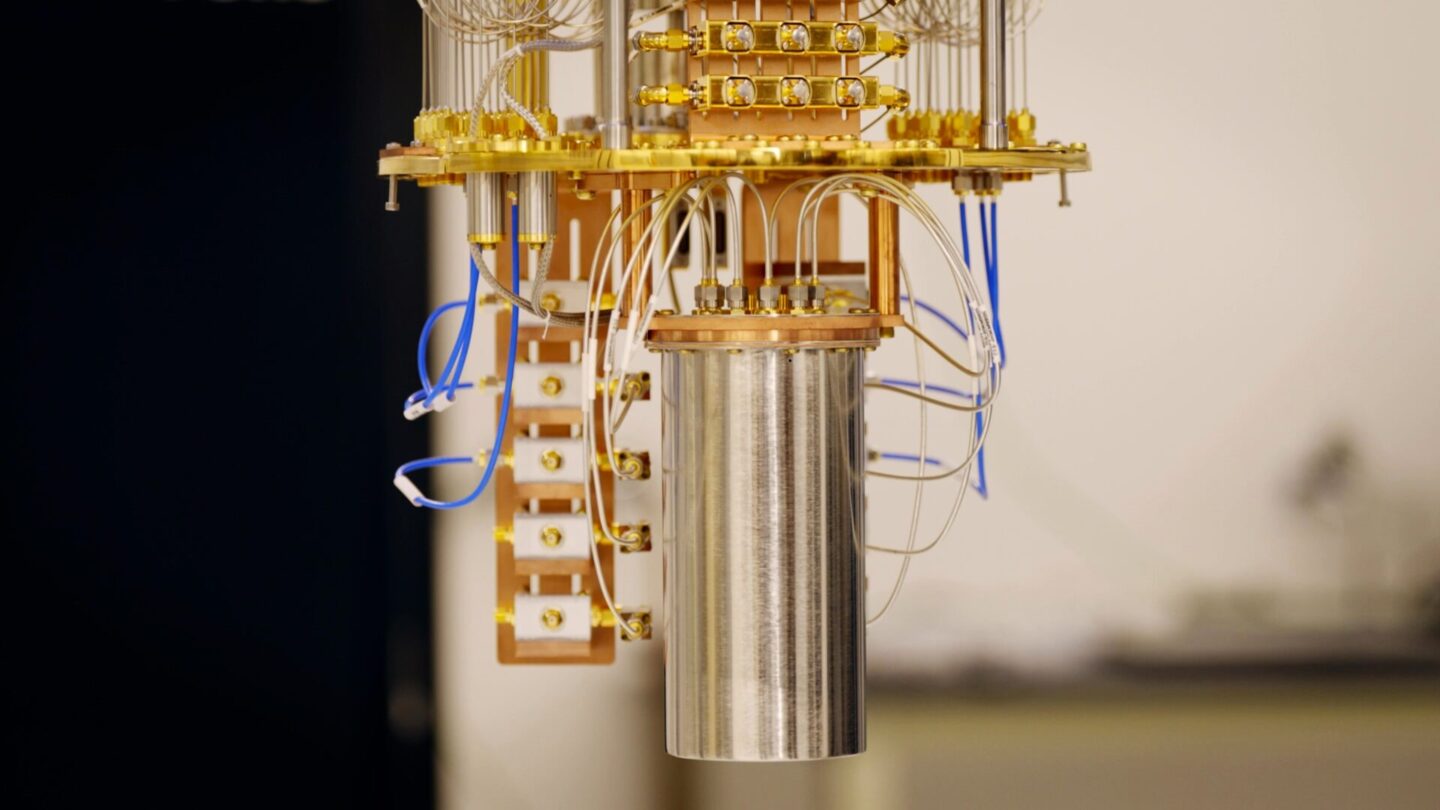
Implications for Quantum Science
The work of Andrei’s research group has broad implications for various fields in quantum science, from quantum computing to quantum metrology and optimization algorithms. “These synthetic platforms can essentially be viewed as quantum machines that generate highly entangled states which can be used in various quantum computing and quantum metrology applications,” Andrei says. “For example, in quantum computing, the key is to demonstrate quantum error correction. The intuition we gain from preparing these many-body states of matter is how to encode quantum information, delocalized across many entangled qubits, in a way that is robust against local errors.”
Capitalizing on these synthetic quantum systems to generate large, entangled states can also be useful for quantum metrology: building sensors with enhanced precision for detecting weak electromagnetic signals that would otherwise be hard to detect using classical resources.
Looking ahead, Andrei sees great potential in hybrid approaches that combine many-body quantum simulators with either quantum or classical computers. “What I’m excited for in the future is exploring ways to merge these technologies. We could develop a hybrid approach that interfaces quantum computers with artificial quantum matter, leading to efficient tools for controlling and probing these materials.”
He adds, “We could also envision hybrid approaches marrying classical computers with these synthetic many-body quantum systems, harnessing their complex quantum dynamics as a resource for solving optimization problems and developing new techniques in machine learning and neuromorphic computing.”
Find out more about the Bluefors LD system and our next generation Gas Handling System powering it.