Detecting Dark Matter: The HAYSTAC experiment at Yale University
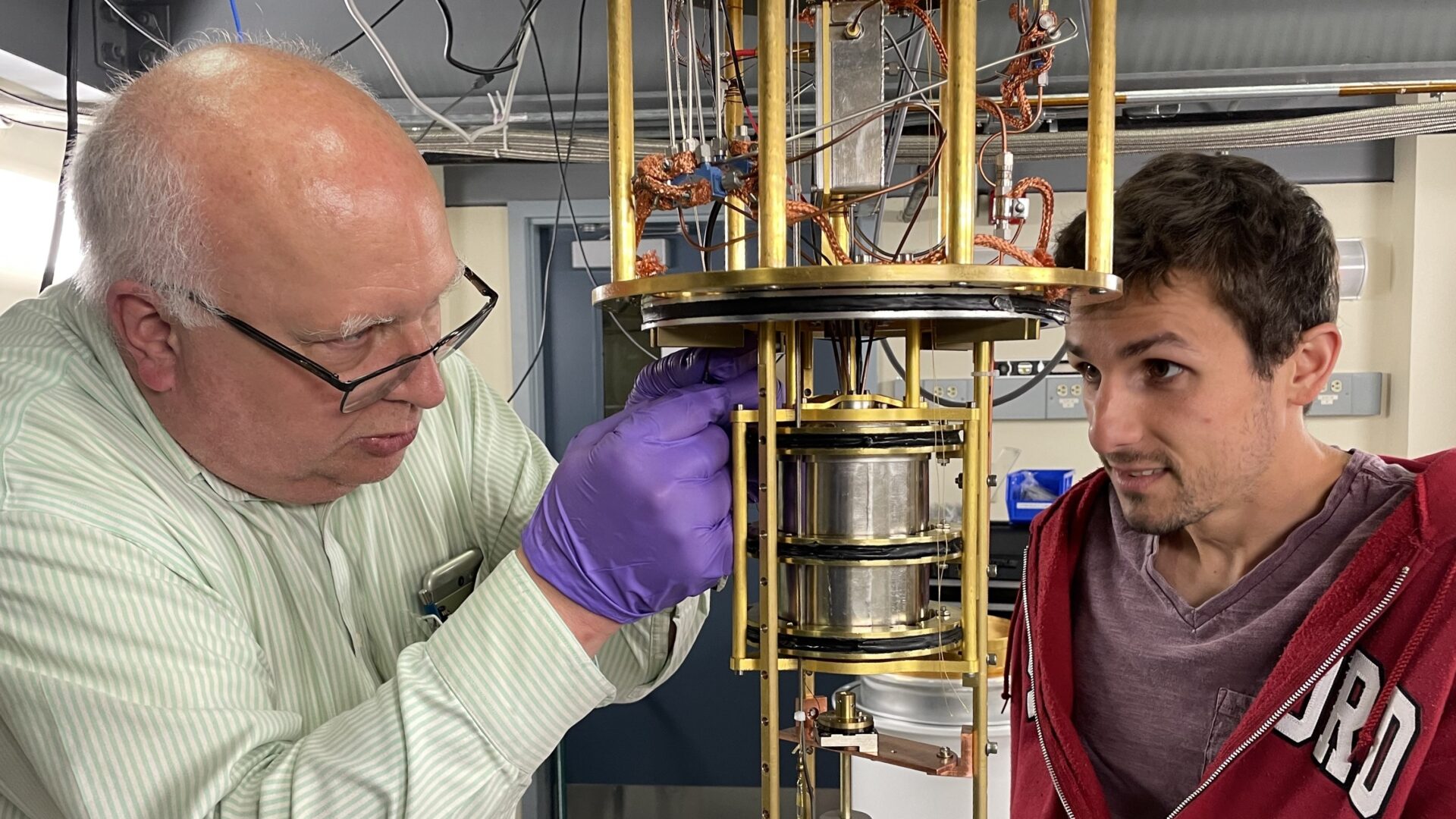
Fundamental physics research helps us better understand the universe and what it contains. The Department of Physics at Yale University develops technologies to explore these questions and investigate nature at the most fundamental level.
Searching for dark matter is like looking for a needle in a near-infinite haystack. But at Yale, there are several ongoing efforts to detect dark matter axions in the galactic halo. One of these projects is the Haloscope At Yale Sensitive To Axion CDM (HAYSTAC) experiment, based on Yale’s campus at Wright Laboratory. This particular project is unique, because it employs techniques from quantum physics research to enhance the experiment’s sensitivity.
Read on to discover what dark matter is, how the HAYSTAC experiment is trying to detect it, and what role Bluefors systems play in the experiment.
What Is Dark Matter?
Dark matter is a type of matter that does not interact with light or electromagnetic fields, making it invisible. It acts as the unseen glue that holds the cosmos together and constitutes most of the mass in galaxies. Dark matter is a fundamental building block for our understanding of the universe, but because it has never been directly detected, its existence has yet to be proved.
Michael Jewell, Associate Research Scientist at the Department of Physics and the Maruyama Lab, is working on the HAYSTAC experiment. He explains the current status of dark matter research:
“The consensus in the physics community is that most of the matter in the universe is ‘dark matter’, or matter which, outside of its gravitational interaction, has at most feeble interactions with normal matter,” Michael explains. “The existence of dark matter is inferred from an array of cosmological/astrophysical observations, such as galaxy rotation curves, that can’t be explained with just the visible matter. While these observations offer substantial evidence for dark matter, direct detection would help offer definitive proof of its existence.”
Detection of such a weakly interacting particle is challenging, and this is made even harder because we do not know what dark matter is made of. “Fortunately, several promising theories have been proposed which are in principle within reach of modern technology,” Michael says.
“One of these candidates is the axion, a particle theorized to solve a completely unrelated problem in particle physics – the Strong-CP problem in quantum chromodynamics (QCD) – but also happens to have all the right properties to be the answer for Dark Matter. The ability to kill two birds with one stone has pushed the axion to the forefront of Dark Matter searches, with several experiments such as HAYSTAC now actively attempting to discover it.”
Mitigating Noise to Search for Axions
To search for cold dark matter (CDM) axions, the HAYSTAC experiment employs quantum-enhanced detection technologies. The experiment is composed of a resonant microwave cavity that is held at an extremely cold temperature, immersed in a large magnetic field, and connected to a microwave amplification chain.
Detecting axions poses a significant challenge due to their weak interaction with normal matter. Axions are incredibly light and have minimal energy, behaving more like waves than particles. “The power expected from axions converting in the detector is around 10^(-24) watts (a yoctowatt) – a minuscule signal easily drowned out by noise,” Michael says.
The sensitivity required to detect such a signal is astounding. Steve Lamoreaux, a Yale physics professor and Principal Investigator for HAYSTAC, described the capabilities of the HAYSTAC detector in a 2017 Yale Scientific Magazine article followingly: “Imagine a match lit on the surface of the Moon. The rate of energy entering the pupil of your eye, when the match is viewed from the Earth, is about the level of sensitivity we achieve.”
Such high sensitivity is only made possible by maintaining an ultra-low noise environment.
Thermal radiation – the photons emitted by matter – is a major source of noise. To minimize its interference and improve axion detection, ultra-low temperatures are essential. “To reduce thermal noise and to have a chance at detecting axions in a reasonable timeframe, the HAYSTAC experiment is operated in a Bluefors LD250 dilution fridge, allowing the system to be cooled and operated at 60mK for months at a time,” Michael explains.
In essence, the HAYSTAC detection device produces a magnetic field that turns axions into photons. The mass of the axion determines the frequency at which these photons oscillate. Therefore, when the detector is tuned in to one specific frequency at a time, it can amplify these oscillations to make them detectable.
The HAYSTAC experiment’s microwave amplification chain utilizes superconducting Josephson Parametric Amplifiers (JPAs ) as key components. These amplifiers, commonly used in quantum information processing, enable the detection of extremely faint signals, by enhancing signal strength while minimizing noise, making them ideal for sensitive measurements. In the HAYSTAC setup, the Bluefors LD system is used to provide the necessary cooling for these sensitive amplifiers.
“The JPAs have been used to achieve sub-quantum limited noise through squeezed vacuum states. This advancement has enabled the HAYSTAC experiment to roughly double its scanning rate, accelerating how quickly it can search for axions across various masses and frequencies, as detailed in Nature 590, 238 (2021),” Michael says.
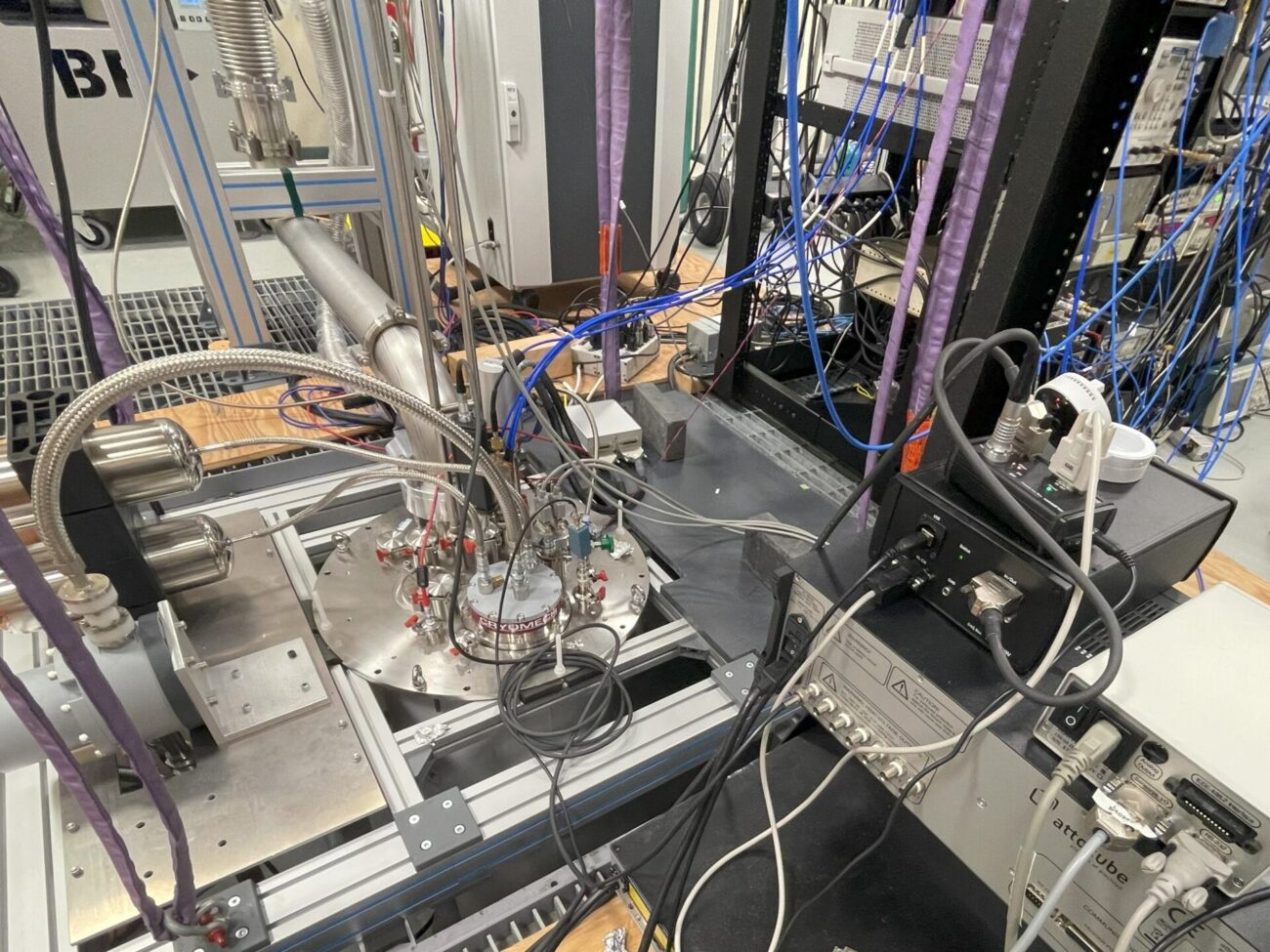
Customized Magnet Setup for Further Sensitivity
In addition to lowering the noise, Michael explains that the sensitivity of the experiment can be improved further with larger magnetic fields and larger cavity volumes. Generating a powerful magnetic field around the cooled microwave cavity is crucial for detecting axions. Therefore, in the HAYSTAC setup, the coldest part of the dilution refrigerator is situated inside a large magnet.
Michael elaborates on the magnet setup of the experiment: “HAYSTAC is currently using a dry 9T superconducting magnet with a 14 cm diameter cold bore from Cryomagnetics. While the magnet is separately cooled by a Cryomech cryocooler, it ultimately shares a cold space with the LD250 system.”
“The cavity itself is thermally linked and supported by the fridge’s Mixing Chamber Plate, hanging into the center of the magnet’s bore. Because the magnet operates at around 4K, we shield the cavity with a thermal shield attached to the fridge’s still plate that extends into the magnet bore and surrounds the cavity. This integrated system has allowed for operation at 60mK in an 8-9T magnetic field for months at a time, improving our chances of detecting axions.”
Because the magnet is an integral element of the experiment, the Bluefors LD250 system was customized to be compatible with it. “We had purchased the Cryomagnetics magnet earlier to mate with the previous dilution refrigerator we had. However, the Bluefors engineers adapted the flanges and thermal shields to fit the existing shields designed for the previous system. This way, it was a perfect plug-in replacement,” Michael says.
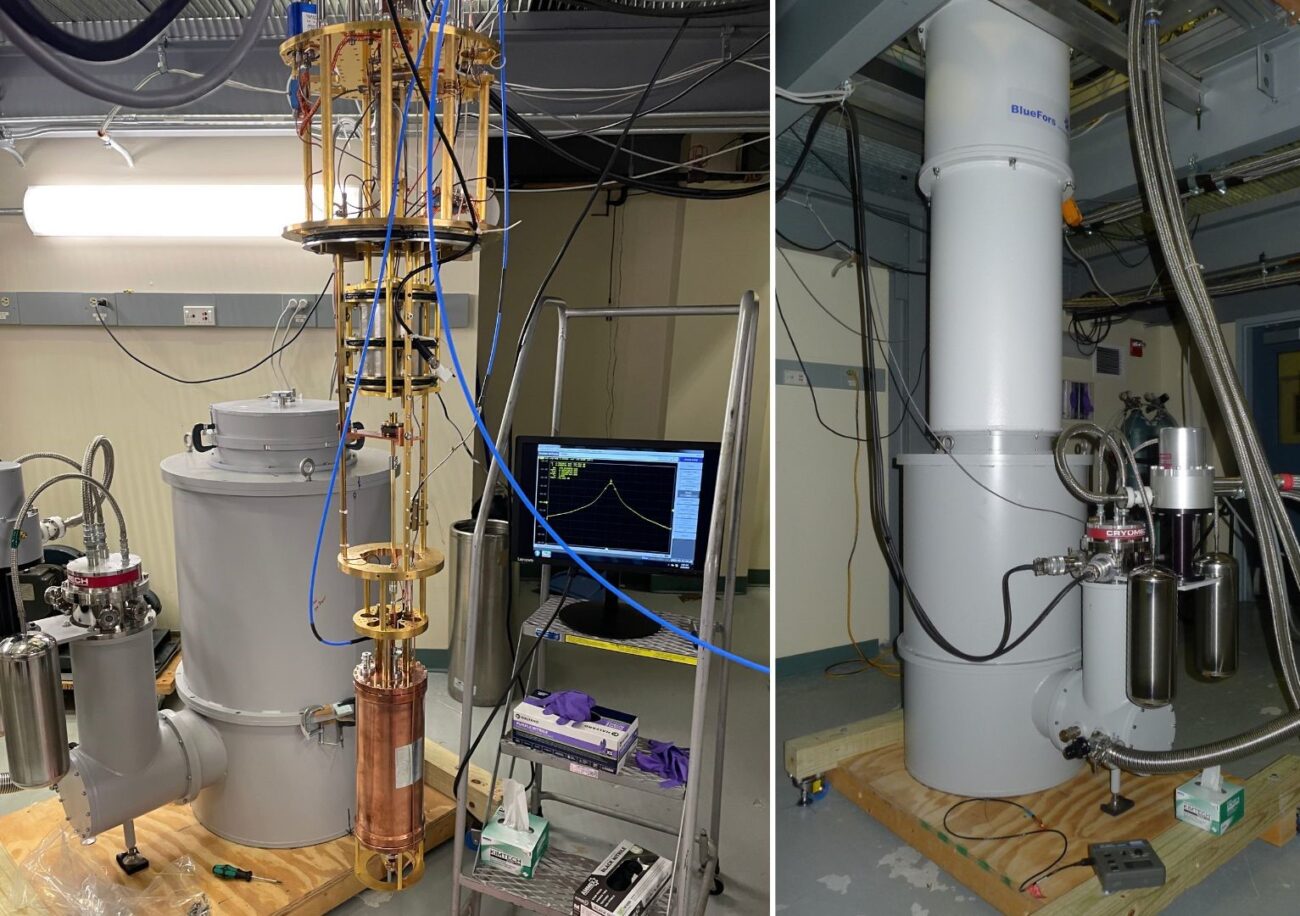
Paving the Way for Dark Matter Discoveries
Dark matter research has come a long way, but many uncertainties remain. “Right now, there are several experiments searching for axions with masses in the approximate range of 1-50ueV (~1-10 GHz), but there is still a significant amount of parameter space outside of this range where the axion could exist,” Michael explains.
Expanding search efforts beyond the current range presents significant challenges, to which quantum technology could be the answer. “A major hurdle is that larger masses (higher frequencies) naturally result in smaller resonators, limiting the number of axions interacting in the detector,” Michael says. “To combat this, we are looking into various quantum sensors that can reduce the system noise and offset the drop in signal power.”
HAYSTAC is at the forefront of these advances, performing the first dark matter search that employed quantum squeezing to achieve sub-quantum limited noise. Michael adds that “several new ideas are being developed which could offer enhancements beyond what has already been employed.”
Reliable Solutions for Cutting-edge Research
Dark matter research is a fascinating example of the diverse applications of Bluefors’ cryogenic measurement systems. Our LD dilution refrigerator is a highly versatile system, capable of supporting numerous experimental needs—including innovative applications such as HAYSTAC—by consistently delivering reliable cooling power at millikelvin temperatures.
Bluefors is committed to supporting researchers in their quest for progress. We provide reliable and innovative solutions, along with the flexibility and collaboration needed to address each research group’s unique needs.
Find out more about the Bluefors LD system and discover the wide range of applications of Bluefors technology.